Metformin, an affordable and safe biguanide derivative, due to its ability to influence metabolism, is widely used as a first-line treatment for type 2 diabetes. The use of metformin has expanded from type 2 diabetes to gestational diabetes, polycystic ovary syndrome, the metabolic syndrome and diabetes prevention (Kooy et al, 2009).
Type 2 diabetes is an increasing public health burden, and managing the disease and its complications accounts for close to 10% of the entire NHS budget in the UK (Sharma et al, 2016). Metformin is the most common first-line medication and mainstay of current treatment in type 2 diabetes following dietary and lifestyle advice. In 2022, more than 89% of patients were initiated on metformin (Sharma et al, 2016).
Metformin hydrochloride is generally considered to be a safe drug, with minimal side effects. However, several prospective studies have shown that it can cause vitamin B12 deficiency (Ting et al, 2006; Kim et al, 2019). Since June 2022, the Department of Health guidelines have recommended screening for B12 deficiency in people on metformin (Davies, 2022).
The clinical significance of metformin-induced vitamin B12 deficiency ranges from milder manifestations, such as fatigue and paraesthesia, to severe features like pancytopenia and degeneration of the spinal cord. Thus, detecting vitamin B12 deficiency and treating it is vital in preventing such complications. In addition, it is entirely possible that symptoms of neuropathy resulting from underlying B12 deficiency have been attributed to diabetic neuropathy.
Physiology of glycaemic control and the role of metformin
One of metformin’s primary effects is the suppression of gluconeogenesis in the liver. Studies have observed that a pharmacological concentration of metformin reduces cyclic adenosine monophosphate (cAMP)-stimulated production of glucose by 58% in cultured primary hepatocytes (Wang et al, 2019). This effect is a result of the metformin-driven suppression of glucagon.
Glucagon, a hormone secreted by alpha-cells in the pancreas, stimulates gluconeogenesis. In hepatocytes, glucagon binding with its receptor stimulates adenylate cyclase responsible for cAMP production. In turn, cAMP activates protein kinase A (PKA) engaged in glucose production. Studies have suggested that metformin inhibits adenylate cyclase as a result of decreasing adenosine triphosphate (ATP) level. In summary, metformin impairs the glucagon signalling pathway in hepatocytes via the inhibition of cAMP formation essential for PKA activation, thereby preventing gluconeogenesis (Foretz et al, 2014; Wang et al, 2019; Szymczak-Pajor et al, 2022).
In addition, metformin diminishes the electron transport chain activity within the mitochondrial respiratory chain complex I, an important pathway required for the synthesis of ATP. With the effect of lowering ATP, the lowered energy charge in hepatocytes leads to suppression of gluconeogenesis (Owen et al, 2000; Szymczak-Pajor et al, 2022). Thus, metformin acts in many pathways to reduce glucose output by decreasing gluconeogenesis.
As well as metformin’s glucose-lowering ability in the liver, the drug has many other effects around the body. It has been shown to increase peripheral glucose uptake (by ~13%), decrease hepatic gluconeogenesis (by ~20%) and reduce fasting plasma glucose levels.
The molecular mechanism by which metformin increases peripheral glucose uptake in muscle cells is associated with the inhibition of SH2-containing inositol 5-phosphatase (SHIP2). This enzyme is responsible for dephosphorylation of phosphatidylinositol 3,4,5-triphosphate to phosphatidylinositol 4,5-bisphosphate. Metformin blocks the activity of SHIP2, thereby decreasing glucose transporters (GLUT) and increasing glucose uptake in skeletal muscle cells (Foretz et al, 2014; Szymczak-Pajor et al, 2022).
People with type 2 diabetes present with abnormal metabolism of lipids, leading to significantly increased coexistence of fatty liver and cardiovascular diseases. However, metformin improves lipid metabolism by decreasing concentrations of chylomicrons in type 2 diabetes. In addition, metformin reduces cholesterol levels in the circulation via a reduction in the reabsorption of bile acids in the intestine and an increase in chylomicron clearance (Geerling et al, 2014).
Overall, metformin has many advantages in the treatment of type 2 diabetes. Through its function as an anti-hyperglycaemic agent, individuals reduce their risk of microvascular and macrovascular complications, such as nephropathy, neuropathy, cardiovascular diseases and strokes.
Metformin pharmacology: Distribution, absorption, bioavailability, metabolism and excretion
Metformin is a glucose-lowering drug that works by reducing hepatic glucose production in both adenosine monophosphate-activated protein kinase (AMPK)-independent and AMPK-dependent pathways (Kooy et al, 2009; Szymczak-Pajor, 2022). After oral ingestion, metformin is absorbed by proximal small-intestine enterocytes via passive and facilitated diffusion (Figure 1). The primary transporter involved in metformin’s absorption is plasma membrane monoamine transporter (PMAT), present on polarised enterocyte apical membranes. Following the transport of metformin through the small intestine, it is absorbed via the organic cation transporter 1 (OCT1) into the portal vein. Several other transporters participating in the intestinal absorption of metformin, such as serotonin transporter (SERT), thiamine transporter 2 (THTR2) and carnitine/organic cation transporter 1 (OCTN1), have also been identified (Kooy et al, 2009; Szymczak-Pajor, 2022).
As a result of its transport in the blood, metformin is delivered directly to the liver, where its uptake is mediated by OCT1 and OCT3, achieving a much higher concentration than in the portal vein. Through the hepatic uptake of metformin, its plasma concentration drops (Szymczak-Pajor, 2022).
Metformin is excreted through the involvement of multidrug and toxin extrusion 1 (MATE1) transporter. MATE1 expressed in hepatocytes is involved in the elimination of the unchanged drug with the bile or in transport of metformin with the blood to the kidney (Foretz et al, 2014; Szymczak-Pajor, 2022). The major clearance pathway is through renal excretion in unchanged form by tubular secretion into the urine. The transport of metformin to the kidney is mediated by many transporters, notably MATE1 and MATE2.
There are three oral dosage forms of metformin: tablets, liquid and extended-release tablets. The liquid is usually taken with meals one or two times a day. The ordinary tablet formulation is usually taken with meals two or three times a day. The extended-release tablet is usually taken once daily with an evening meal (Scheen and Paquot, 2013; Geerling et al, 2014).
Despite its overall safety profile, metformin does have a few side effects. A typical adverse effect of metformin therapy is now recognised as decreased vitamin B12 levels, or vitamin B12 deficiency – a clinically important and treatable condition. This is especially true for people receiving greater doses and those on metformin for longer periods of time (de Jager et al, 2010).
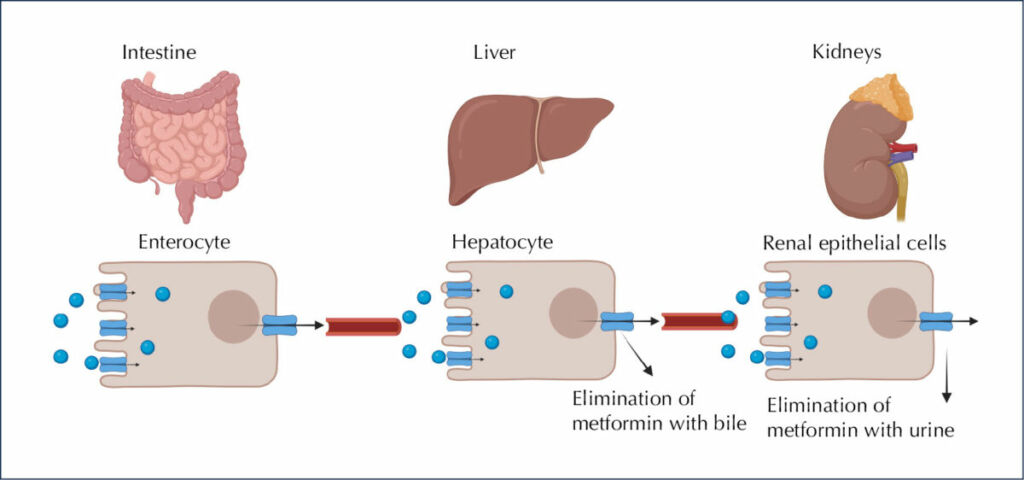
Figure 1. Fate of metformin in the body. After ingestion, 50% of metformin is absorbed from passive and facilitated diffusion in intestinal enterocytes. Then, the drug travels via the portal vein and eventually is excreted by the kidneys, in the unchanged form, with urine. Created using BIORENDER. Adapted from Szymczak-Pajor et al, 2022.
Pathophysiology of metformin and B12 deficiency
Vitamin B12 is a water-soluble vitamin, commonly known as cobalamin. Vitamin B12 supports the health of the body’s nerve and blood cells. It is primarily found in dairy products, such as milk and cheese, and in eggs (Green et al, 2017; Ankar and Kumar, 2022).
Once ingested, vitamin B12 is released from its food-carrier proteins by proteolysis, and binds to a glycoprotein called haptocorrin. In the duodenum, the free vitamin B12 binds to intrinsic factor (IF), a glycoprotein secreted by gastric parietal cells, resulting in the formation of an IF–vitamin B12 complex. The IF–vitamin B12 complex subsequently binds, in a calcium-dependent manner, to the cubilin receptor on the enterocytes of the distal ileum, resulting in the absorption of vitamin B12 by receptor-mediated endocytosis.
Vitamin B12 is released from its receptors and, after degradation by lysosomes, the IF-vitamin B12 complex is released from its receptor, IF is degraded in lysosomes and vitamin B12 enters the circulation via the multidrug resistance protein 1 (MDR1) transporter. In the circulation, approximately 20%–25% of vitamin B12 is bound to its binding protein, transcobalamin. The remaining 75%–80% of vitamin B12 is stored in the liver (Green et al, 2017).
Vitamin B12 functions as a cofactor for enzymes that catalyse the conversion of homocysteine into methionine, which is then converted into intermediates that are used in the synthesis of pyrimidine bases of DNA.
Therefore, vitamin B12 deficiency leads to homocysteine accumulation, impaired DNA synthesis and haematological abnormalities, such as formation of hyper-segmented neutrophils.
The clinical significance of vitamin B12 lies in megaloblastic anaemia, a condition in which the bone marrow produces abnormally large, structurally abnormal and immature red blood cells owing to ineffective haemopoiesis. Anaemia can be associated with various non-specific symptoms, including pallor, palpitations, tachycardia and fatigue. Although megaloblastic anaemia is a common presentation, some patients may also present with neuropathy or can be asymptomatic. Other symptoms of low vitamin B12 levels may include mental disturbance, depression, irritability, cognitive impairment, glossitis, mouth ulcers, and visual and motor disturbances (Dali-Youcef and Andrès, 2009; Green et al, 2017).
The mechanism by which metformin reduces vitamin B12 levels remains unclear, but it is most likely to be multifactorial. Some of the proposed mechanisms for metformin-induced vitamin B12 deficiency include:
- Interference with calcium-dependent binding of the IF-vitamin B12 to the cubilin receptor on enterocytes at the ileum level.
- Alteration in small intestine motility, leading to bacterial overgrowth and subsequent inhibition of IF–vitamin B12 complex absorption in the distal ileum.
- Alteration in bile acid metabolism and reabsorption, resulting in impaired enterohepatic circulation of vitamin B12.
- Increased liver accumulation of vitamin B12, resulting in altered tissue distribution and metabolism of vitamin B12.
- Reduced IF secretion by gastric parietal cells.
Amongst these mechanisms, metformin’s ability to interfere with the calcium-dependent membranes responsible for vitamin B12 intrinsic factor absorption has been the most accepted (Dali-Youcef and Andrès, 2009; Green et al, 2017; Szymczak-Pajor et al, 2022.
How a specialist diabetes service improved outcomes for people with diabetes on dialysis.
1 Nov 2024